Anatomy and Physiology of the Eye
The eyeball, which is an elongated sphere about 2.5 cm in diameter, has three layers, or coats: the sclera, the choroid, and the retina (Fig. 9.7). Only the retina contains photoreceptors for light energy. Table 9.1 gives the functions of the parts of the eye.
The outer layer, the sclera, is white and fibrous except for the cornea, which is made of transparent collagen fibers. The cornea is the window of the eye. The middle, thin, darkly pigmented layer, the choroid, is vascular and absorbs stray light rays that photoreceptors have not absorbed. Toward the front, the choroid becomes the donut-shaped iris. The iris regulates the size of the pupil, a hole in the center of the iris through which light enters the eyeball. The color of the iris (and therefore the color of your eyes) correlates with its pigmentation. Heavily pigmented eyes are brown, while lightly pigmented eyes are green or blue. Behind the iris, the choroid thickens and forms the circular ciliary body. The ciliary body contains the ciliary muscle, which controls the shape of the lens for near and far vision.
The lens, attached to the ciliary body by ligaments, divides the eye into two compartments; the one in front of the lens is the anterior compartment, and the one behind the lens is the posterior compartment. The anterior compartment is filled with a clear, watery fluid called the aqueous humor. A small amount of aqueous humor is continually produced each day.
Figure 9.7 Anatomy of the human eye. Notice that the sclera, the outer layer of the eye, becomes the cornea and that the choroid, the middle layer, is continuous with the ciliary body and the iris. The retina, the inner layer, contains the photoreceptors for vision; the fovea centralis is the region where vision is most acute.

Normally, it leaves the anterior compartment by way of tiny ducts. When a person has glaucoma, these drainage ducts are blocked, and aqueous humor builds up. If glaucoma is not treated, the resulting pressure compresses the arteries that serve the nerve fibers of the retina, where photoreceptors are located. The nerve fibers begin to die due to lack of nutrients, and the person becomes partially blind. Eventually, total blindness can result.
The third layer of the eye, the retina, is located in the posterior compartment, which is filled with a clear, gelatinous material called the vitreous humor. The retina contains photoreceptors called rod cells and cone cells. The rods are very sensitive to light, but they do not see color; therefore, at night or in a darkened room, we see only shades of gray. The cones, which require bright light, are sensitive to different wavelengths of light, and therefore we have the ability to distinguish colors. The retina has a very special region called the fovea centralis where cone cells are densely packed. Light is normally focused on the fovea when we look directly at an object. This is helpful because vision is most acute in the fovea centralis. Sensory fibers from the retina form the optic nerve, which takes nerve impulses to the brain.

Function of the Lens
The lens, assisted by the cornea and the humors, focuses images on the retina (Fig. 9.8a). Focusing starts with the cornea and continues as the rays pass through the lens and the humor. The image produced is much smaller than the object because light rays are bent (refracted) when they are brought into focus.
Notice that the image on the retina is inverted (upside down) and reversed from left to right. The shape of the lens is controlled by the ciliary muscle within the ciliary body. When we view a distant object, the ciliary muscle is relaxed, causing the suspensory ligaments attached to the ciliary body to be taut; therefore, the lens remains relatively flat (Fig. 9.8b). When we view a near object, the ciliary muscle contracts, releasing the tension on the suspensory ligaments, and the lens rounds up due to its natural elasticity (Fig. 9.8c). If the eyeball is too long or too short, the person may need corrective lenses to focus the image on the retina.
Figure 9.8 Focusing. a. Light rays from each point on an object are bent by the cornea and the lens in such a way that an inverted and reversed image of the object forms on the retina. b. When focusing on a distant object, the lens is flat because the ciliarymuscle is relaxed and the suspensory ligament is taut. c. When focusing on a near object, the lens accommodates; it becomes rounded because the ciliarymuscle contracts, causing the suspensory ligament to relax.
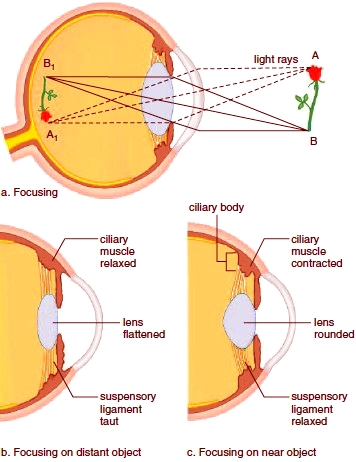
Accommodation: It is said that visual accommodation must occur for close vision. Because close work requires contraction of the ciliary muscle, it very often causes muscle fatigue, known as eyestrain. Usually after the age of 40, the lens loses some of its elasticity and is unable to accommodate. Bifocal lenses may then be necessary for those who already have corrective lenses.
Vision Pathway
The pathway for vision begins once light has been focused on the photoreceptors in the retina. Some integration occurs in the retina where nerve impulses begin before the optic nerve transmits them to the brain.
Function of Photoreceptors: Figure 9.9 illustrates the structure of the photoreceptors called rod cells and cone cells. Both rods and cones have an outer segment joined to an inner segment by a stalk. Pigment molecules are embedded in the membrane of the many disks present in the outer segment. Synaptic vesicles are located at the synaptic endings of the inner segment. The visual pigment in rods is a deep purple pigment called rhodopsin. Rhodopsin is a complex molecule made up of the protein opsin and a light-absorbing molecule called retinal, which is a derivative of vitamin A. When a rod absorbs light, rhodopsin splits into opsin and retinal, leading to a cascade of reactions and the closure of ion channels in the rod cell’s plasma membrane. The release of inhibitory transmitter molecules from the rod’s synaptic vesicles ceases. Thereafter, nerve impulses go to the visual area of the cerebral cortex. Rods are very sensitive to light and therefore are suited to night vision. (Because carrots are rich in vitamin A, it is true that eating carrots can improve your night vision.) Rod cells are plentiful throughout the entire retina; therefore, they also provide us with peripheral vision and perception of motion.
The cones, on the other hand, are located primarily in the fovea and are activated by bright light. They allow us to detect the fine detail and the color of an object. Therefore, the condition called macular degeneration, which affects the fovea, is particularly devastating. The What’s New reading on page 176 describes the condition and tells of a promising treatment that may soon be available.
Color vision depends on three different kinds of cones, which contain pigments called the B (blue), G (green), and R (red) pigments. Each pigment is made up of retinal and opsin, but there is a slight difference in the opsin structure of each, which accounts for their individual absorption patterns. Various combinations of cones are believed to be stimulated by in-between shades of color.
Figure 9.9 Photoreceptors in the eye. The outer segment of rods and cones contains stacks ofmembranous disks, which contain visual pigments. In rods, the membrane of each disk contains rhodopsin, a complex molecule containing the protein opsin and the pigment retinal. When retinal absorbs light energy, it splits, releasing opsin, which sets in motion a cascade of reactions that cause ion channels in the plasma membrane to close. Thereafter, nerve impulses go to the brain.

Function of the Retina: The retina has three layers of neurons (Fig. 9.10). The layer closest to the choroid contains the rod cells and cone cells; the middle layer contains bipolar cells; and the innermost layer contains ganglion cells, whose sensory fibers become the optic nerve. Only the rod cells and the cone cells are sensitive to light, and therefore light must penetrate to the back of the retina before they are stimulated. The rod cells and the cone cells synapse with the bipolar cells, which in turn synapse with ganglion cells that initiate nerve impulses. Notice in Figure 9.10 that there are many more rod cells and cone cells than ganglion cells. In fact, the retina has as many as 150 million rod cells and 6 million cone cells but only one million ganglion cells. The sensitivity of cones versus rods is mirrored by how directly they connect to ganglion cells. As many as 150 rods may activate the same ganglion cell. No wonder stimulation of rods results in vision that is blurred and indistinct. In contrast, some cone cells in the fovea centralis activate only one ganglion cell. This explains why cones, especially in the fovea, provide us with a sharper, more detailed image of an object.
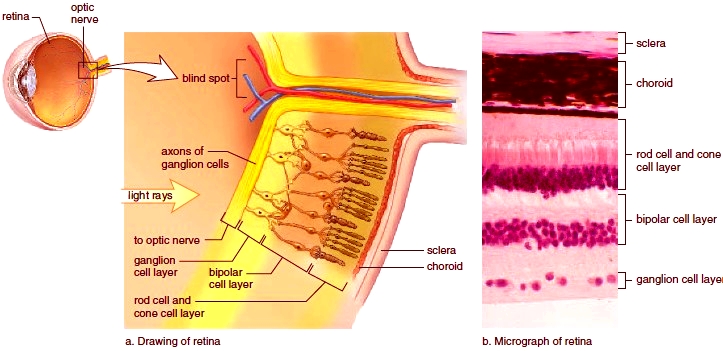
Figure 9.10 Structure and function of the retina. a. The retina is the inner layer of the eyeball. Rod cells and cone cells, located at the back of the retina nearest the choroid, synapse with bipolar cells, which synapse with ganglion cells. Integration of signals occurs at these synapses; therefore, much processing occurs in bipolar and ganglion cells. Further, notice thatmany rod cells share one bipolar cell, but cone cells do not. Certain cone cells synapse with only one bipolar cell. Cone cells, in general, distinguish more detail than do rod cells. b. This micrograph shows that the sclera and choroid are relatively thin compared to the retina, which is composed of several layers of cells.
As signals pass to bipolar cells and ganglion cells, integration occurs. Each ganglion cell receives signals from rod cells covering about one square millimeter of retina (about the size of a thumbtack hole). This region is the ganglion cell’s receptive field. Some time ago, scientists discovered that a ganglion cell is stimulated only by nerve impulses received from the center of its receptive field; otherwise, it is inhibited. If all the rod cells in the receptive field receive light, the ganglion cell responds in a neutral way-that is, it reacts only weakly or perhaps not at all. This supports the hypothesis that considerable processing occurs in the retina before nerve impulses are sent to the brain. Additional integration occurs in the visual areas of the cerebral cortex. Blind Spot Figure 9.10 provides an opportunity to point out that there are no rods and cones where the optic nerve exits the retina. Therefore, no vision is possible in this area. You can prove this to yourself by putting a dot to the right of center on a piece of paper. Use your right hand to move the paper slowly toward your right eye while you look straight ahead. The dot will disappear at one point-this is your blind spot.
From the Retina to the Visual Cortex: As stated, sensory fibers from the ganglion cells in the retina assemble to form the optic nerves. The optic nerves carry nerve impulses from the eyes to the optic chiasma. The optic chiasma has an Xshape formed by a crossing over of some of the optic nerve fibers. At the chiasma, fibers from the right half of each retina converge and continue on together in the right optic tract, and fibers from the left half of each retina converge and continue on together in the left optic tract.
The optic tracts sweep around the hypothalamus, and most fibers synapse with neurons in nuclei (masses of neuron cell bodies) in the thalamus. Axons from the thalamic nuclei form optic radiations that take nerve impulses to the primary visual areas of the occipital lobes (Fig. 9.11). The occipital lobes are a part of the cerebral cortex.
The visual cortex consists of the primary visual area and the visual association areas of the occipital lobes. Notice that the image arriving at the thalamus, and therefore the primary visual areas, has been split because the left optic tract carries information about the right portion of the visual field and the right optic tract carries information about the left portion of the visual field. Therefore, the right and left visual cortex must communicate with each other for us to see the entire visual field. Also, because the image is inverted and reversed (see Figs. 9.8 and 9.11) it must be righted for us to correctly perceive the visual field.
The most surprising finding has been that each primary visual area of the cerebral cortex acts like a post office, parceling out information regarding color, form, motion, and possibly other attributes to different portions of the adjoining visual association areas. In other words, the visual field has been taken apart even though we see a unified field. The visual association areas are believed to rebuild the field and give us an understanding of it.
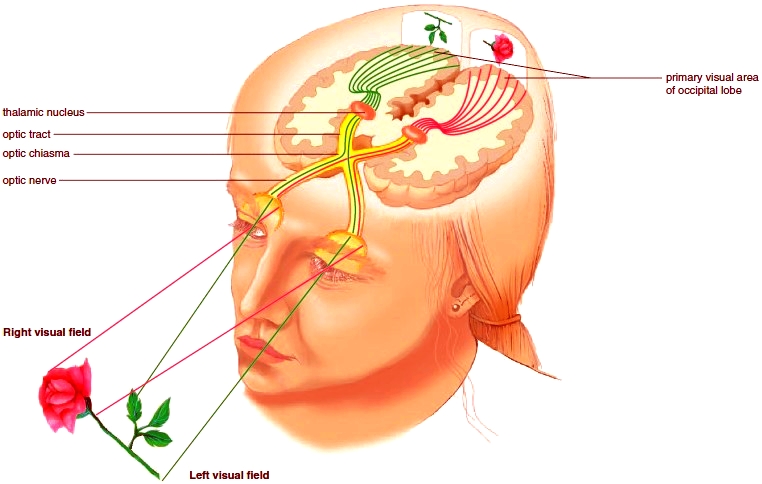
Figure 9.11 Optic chiasma. Both eyes “see” the entire visual field. Because of the optic chiasma, data from the right half of each retina go to the right visual area of the cerebral cortex, and data from the left half of the retina go to the left visual area of the cerebral cortex. These data are then combined to allow us to see the entire visual field. Note that the visual pathway to the brain includes the thalamus, which has the ability to filter sensory stimuli.