Nerve Impulses
When axons are resting, they are not conducting nerve impulses. When they are active, axons are conducting nerve impulses, also called action potentials.
Resting Potential
When an axon is resting, its membrane is polarized; that is, the outside is positive compared to the inside, which is negative. A protein carrier in the membrane, called the sodiumpotassium pump, pumps sodium (Na+) out of the axon and potassium (K+) into the axon. Another factor that causes the inside of the axon to be negative compared to the outside is the presence of large, negatively charged protein ions inside an axon. The polarity across an axon that is not conducting nerve impulses is called the resting potential (Fig. 8.3a).
Action Potential
When the nerve fiber is conducting a nerve impulse (action potential), a change in polarity occurs across the axon’s membrane (Fig. 8.3b). First, the inside of an axon becomes positive compared to the outside (this is called depolarization), and then the inside becomes negative again (this is called repolarization). An action potential requires two types of channels in the membrane: One channel can allow Na+ ions to pass through the membrane, and the other can allow K+ ions to pass through the membrane. During depolarization, Na+ ions move to the inside of the axon, and during repolarization, K+ ions move to the outside.
Figure 8.3 Resting and action potentials in a nonmyelinated axon. a. Resting potential. There are many more Na+ ions outside the axon and many more K+ ions inside the axon. Also, the inside is negative compared to the outside. b. Action potential. First, Na+ gates open, and Na+ ionsmove to the inside of an axon. This causes the inside to become positive. Second, K+ gates open, and K+ ionsmove to the outside. This causes the inside to become negative again.
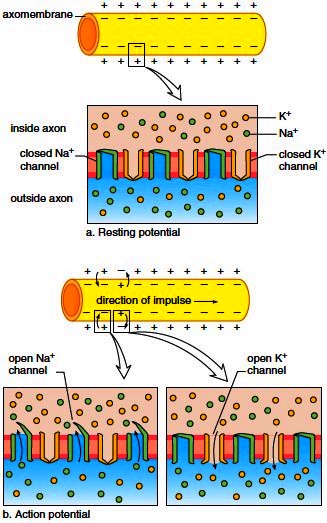
Conduction of Action Potentials
If an axon is unmyelinated, an action potential at one locale stimulates an adjacent part of the axomembrane to produce an action potential. In myelinated fibers, an action potential at one node of Ranvier causes an action potential at the next node (Fig. 8.4). This type of conduction, called saltatory conduction, is much faster than otherwise. In thin, unmyelinated axons, the action potential travels about 1.0 m/sec, and in thick, myelinated fibers, the rate is more than 100 m/sec. The conduction of a nerve impulse (action potential) is an all-or-none event; that is, either an axon conducts a nerve impulse or it does not. The intensity of a message is determined by how many nerve impulses are generated within a given time span. A fiber can conduct a volley of nerve impulses because only a small number of ions are exchanged with each impulse. As soon as an impulse has passed by each successive portion of an axon, it undergoes a short refractory period during which it is unable to conduct an impulse. This ensures the one-way direction of an impulse from cell body to axon terminal. It is interesting to observe that all functions of the nervous system, from our deepest emotions to our highest reasoning abilities, are dependent on the conduction of nerve impulses.

Figure 8.4 Conduction of an action potential in a myelinated axon. The action potential jumps from one neurofibril node to the next along the axon. Thismakes the speed of a nerve impulse much faster than in unmyelinated axons. Almost all axons are myelinated in humans.
Transmission Across a Synapse
Every axon branches into many fine endings, each tipped by a small swelling called an axon terminal. Each swelling lies very close to either the dendrite or the cell body of another neuron. This region of close proximity is called a synapse (Fig. 8.5). At a synapse, the membrane of the first neuron is called the presynaptic membrane, and the membrane of the next neuron is called the postsynaptic membrane. The small gap between is the synaptic cleft. Transmission across a synapse is carried out by molecules called neurotransmitters, which are stored in synaptic vesicles in the axon terminals. When nerve impulses traveling along an axon reach an axon terminal, channels for calcium ions (Ca2+) open, and calcium enters the terminal. This sudden rise in Ca2+ stimulates synaptic vesicles to merge with the presynaptic membrane, and neurotransmitter molecules are released into the synaptic cleft. They diffuse across the cleft to the postsynaptic membrane, where they bind with specific receptor proteins. Depending on the type of neurotransmitter and the type of receptor, the response of the postsynaptic neuron can be toward excitation or toward inhibition. After excitatory neurotransmitters combine with a receptor, a sodium ion channel opens, and Na+ enters the neuron (Fig. 8.5). Other neurotransmitters have an inhibitory effect as described in the next section.
Synaptic Integration
A single neuron can have many dendrites plus the cell body, and both can synapse with many other neurons. Typically, a neuron is on the receiving end of many excitatory and inhibitory signals. An excitatory neurotransmitter produces a potential change called a signal that drives the polarity of a neuron closer to an action potential; an inhibitory neurotransmitter produces a signal that drives the polarity of a neuron farther from an action potential. Excitatory signals have a depolarizing effect, and inhibitory signals have a hyperpolarizing effect. Neurons integrate these incoming signals.
Integration is the summing up of excitatory and inhibitory signals. If a neuron receives many excitatory signals (either from different synapses or at a rapid rate from one synapse), chances are, the axon will transmit a nerve impulse. On the other hand, if a neuron receives both inhibitory and excitatory signals, the summing up of these signals may prohibit the axon from firing.
Neurotransmitter Molecules
At least 25 different neurotransmitters have been identified, but two very well-known ones are acetylcholine (ACh) and norepinephrine (NE). Once a neurotransmitter has been released into a synaptic cleft and has initiated a response, it is removed from the cleft. In some synapses, the postsynaptic membrane contains enzymes that rapidly inactivate the neurotransmitter. For example, the enzyme acetylcholinesterase (AChE) breaks down acetylcholine. In other synapses, the presynaptic membrane rapidly reabsorbs the neurotransmitter, possibly for repackaging in synaptic vesicles or for molecular breakdown. The short existence of neurotransmitters at a synapse prevents continuous stimulation (or inhibition) of postsynaptic membranes.

Figure 8.5 Synapse structure and function. Transmission across a synapse from one neuron to another occurs when a neurotransmitter is released at the presynaptic membrane, diffuses across a synaptic cleft, and binds to a receptor in the postsynaptic membrane.