Physiology of Circulation
Circulation is the movement of blood through blood vessels, from the heart and then back to the heart. In this section, we discuss various factors affecting circulation.
Velocity of Blood Flow
The velocity of blood flow is slowest in the capillaries. What might account for this? Consider that the aorta branches into the other arteries, and these in turn branch into the arterioles, and so forth until blood finally flows into the capillaries. Each time an artery branches, the total cross-sectional area of the blood vessels increases, reaching the maximum cross-sectional area in the capillaries (Fig. 12.12). The slow rate of blood flow in the capillaries is beneficial because it allows time for the exchange of gases in pulmonary capillaries and for the exchange of gases and nutrients for wastes in systemic capillaries.
Conversely, blood flow increases as venules combine to form veins, and velocity is faster in the venae cavae than in the smaller veins. The cross-sectional area of the two venae cavae is more than twice that of the aorta, and the velocity of the blood returning to the heart remains low compared to the blood leaving the heart.
In a resting individual, it takes only a minute for a drop of blood to go from the heart to the foot and back again to the heart! Blood pressure causes blood flow because blood always flows from a higher to a lower pressure difference.
Blood Pressure
Blood pressure is the force of blood against a blood vessel wall. You would expect blood pressure to be highest in the aorta. Why? Because the pumping action of the heart forces blood into the aorta. Further, Figure 12.13 shows that systemic blood pressure decreases progressively with distance from the left ventricle. Blood pressure is lowest in the venae cavae because they are farthest from the left ventricle.
Note also in Figure 12.13 that blood pressure fluctuates in the arterial system between systolic blood pressure and diastolic blood pressure. Certainly, we can correlate this with the action of the heart. During systole, the left ventricle is pumping blood out of the heart, and during diastole the left ventricle is resting.
More important than the systolic and diastolic pressure is the mean arterial blood pressure (MABP). What might determine MABP? One factor is cardiac output (CO). In other words, the greater the amount of blood leaving the left ventricle, the greater the pressure of blood against the wall of an artery. Another factor that determines blood pressure is peripheral resistance, which is the friction between blood and the walls of a blood vessel. All things being equal, the smaller the blood vessel, the greater the resistance and the higher the blood pressure. Similarly, total blood vessel length increases blood pressure because a longer vessel offers greater resistance. An obese person is apt to have high blood pressure because about 200 miles of additional blood vessels develop for each extra pound of fat.
Figure 12.12 Velocity of blood flow changes throughout the systemic circuit. Velocity changes according to the total crosssectional area of vessels.
Figure 12.13 Blood pressure changes throughout the systemic circuit. Blood pressure decreases with distance from the left ventricle.
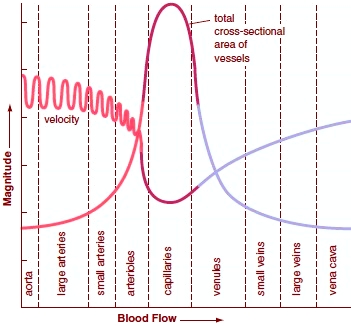

Cardiac Output
The heart rate and the stroke volume determine CO. We learned that the heartbeat is intrinsic but is under extrinsic (nervous) control. Therefore, it can speed up. The faster the heart rate, the greater the blood pressure (assuming constant peripheral resistance). Similarly, the larger the stroke volume, the greater the blood pressure. However, stroke volume and heart rate increase blood pressure only if the venous return is adequate.
Venous Return Venous return depends on three factors:
1. a blood pressure difference-blood pressure is about 16 mm Hg in venules versus 0 mm Hg in the right atrium;
2. the skeletal muscle pump and the respiratory pump, both of which are effective because of the presence of valves in veins;
3. total blood volume in the cardiovascular system.
The skeletal muscle pump works like this: When skeletal muscles contract, they compress the weak walls of the veins. This causes the blood to move past a valve (Fig. 12.14). Once past the valve, backward pressure of blood closes the valve and prevents its return.
The respiratory pump works like this: When inhalation occurs, thoracic pressure falls and abdominal pressure rises as the chest expands. This aids the flow of venous blood back to the heart because blood flows in the direction of reduced pressure. During exhalation, the pressure reverses, but the valves in the veins prevent backward flow.
As you might suspect, gravity can assist the return of venous blood from the head to the heart but not the return of blood from the extremities and trunk to the heart. The importance of the skeletal muscle pump in maintaining CO and blood pressure can be demonstrated by forcing a person to stand rigidly still for a number of hours. Frequently, the person faints because blood collects in the limbs, robbing the brain of oxygen. In this case, fainting is beneficial because the resulting horizontal position aids in getting blood to the head.
As stated, the amount of venous return also depends on the total blood volume in the cardiovascular system. As you know, this volume in the pulmonary circuit and the systemic circuit is 5 L. If this amount of blood decreases, say due to hemorrhaging, blood pressure falls. On the other hand, if blood volume increases (due to water retention, for example), blood pressure rises.

Figure 12.14 Skeletal muscle pump. a. When skeletal muscles contract and compress a vein, blood is squeezed past a valve. b. When muscles relax, the backward flow of blood closes the valve.
Peripheral Resistance
The nervous system and the endocrine system both affect peripheral resistance.
Neural Regulation of Peripheral Resistance A vasomotor center in the medulla oblongata controls vasoconstriction. This center is under the control of the cardioregulatory center. If blood pressure falls, baroreceptors in the blood vessels signal the cardioregulatory center. Thereafter, impulses conducted along sympathetic nerve fibers cause the heart rate to increase and the arterioles to constrict via the vasomotor center. The result is a rise in blood pressure. What factors lead to a reduction in blood pressure? If blood pressure rises above normal, the baroreceptors signal the cardioregulatory center in the medulla oblongata. Subsequently, the heart rate decreases and the arterioles dilate.
Nervous control of blood vessels also causes blood to be shunted from one area of the body to another. During exercise, arteries in the viscera and skin are more constricted than those in the muscles. Therefore, blood flow to the muscles increases. Also, dilation of the precapillary sphincters in muscles means that blood will flow to the muscles and not to the viscera.
Hormonal Regulation of Peripheral Resistance Certain hormones cause blood pressure to rise. Epinephrine and norepinephrine increase the heart rate, as previously mentioned.
When the blood volume and blood sodium level are low, the kidneys secrete the enzyme renin. Renin converts the plasma protein angiotensinogen to angiotensin I, which is changed to angiotensin II by a converting enzyme found in the lungs. Angiotensin II stimulates the adrenal cortex to release aldosterone. The effect of this system, called the renin-angiotensin-aldosterone system, is to raise the blood volume and pressure in two ways. First, angiotensin II constricts the arterioles directly, and second, aldosterone causes the kidneys to reabsorb sodium. When the blood sodium level rises, water is reabsorbed, and blood volume and pressure are maintained.
Two other hormones play a role in the homeostatic maintenance of blood volume. Antidiuretic hormone (ADH) helps increase blood volume by causing the kidneys to reabsorb water. Also, when the atria of the heart are stretched due to increased blood volume, cardiac cells release a hormone called atrial natriuretic hormone (ANH), which inhibits renin secretion by the kidneys and aldosterone secretion by the adrenal cortex. The effect of ANH, therefore, is to cause sodium excretion-that is, natriuresis. When sodium is excreted, so is water, and therefore blood volume and blood pressure decrease (Fig. 12.15).
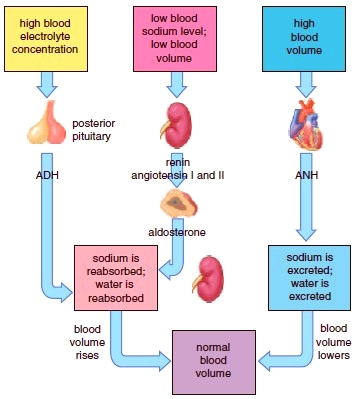
Figure 12.15 Blood volume maintenance. Normal blood volume ismaintained by ADH (antidiuretic hormone) and aldosterone, whose actions raise blood volume, and by ANH (atrial natriuretic hormone), whose actions lower blood volume.
Evaluating Circulation
Taking a patient’s pulse and blood pressure are two ways to evaluate circulation.
Pulse
The surge of blood entering the arteries causes their elastic walls to stretch, but then they almost immediately recoil. This alternating expansion and recoil of an arterial wall can be felt as a pulse in any artery that runs close to the body’s surface, termed pulse points (Fig. 12.16). It is customary to feel the pulse by placing several fingers on the radial artery, which lies near the outer border of the palm side of a wrist. The common carotid artery, on either side of the trachea in the neck, is another accessible location for feeling the pulse. Normally, the pulse rate indicates the rate of the heartbeat because the arterial walls pulse whenever the left ventricle contracts. The pulse is usually 70 times per minute, but can vary between 60 and 80 times per minute.
Blood Pressure
Blood pressure is usually measured in the brachial artery with a sphygmomanometer, an instrument that records changes in terms of millimeters (mm) of mercury (Fig. 12.17). A blood pressure cuff connected to the sphygmomanometer is wrapped around the patient’s arm, and a stethoscope is placed over the brachial artery. The blood pressure cuff is inflated until no blood flows through it; therefore, no sounds can be heard through the stethoscope. The cuff pressure is then gradually lowered. As soon as the cuff pressure declines below systolic pressure, blood flows through the brachial artery each time the left ventricle contracts. The blood flow is turbulent below the cuff. This turbulence produces vibrations in the blood and surrounding tissues that can be heard through the stethoscope. These sounds are called Korotkoff sounds, and the cuff pressure at which the Korotkoff sounds are heard the first time is the systolic pressure. As the pressure in the cuff is lowered still more, the Korotkoff sounds change tone and loudness. When the cuff pressure no longer constricts the brachial artery, no sound is heard. The cuff pressure at which the Korotkoff sounds disappear is the diastolic pressure.
Normal resting blood pressure for a young adult is 120/80. The higher number is the systolic pressure, the pressure recorded in an artery when the left ventricle contracts. The lower number is the diastolic pressure, the pressure recorded in an artery when the left ventricle relaxes.
It is estimated that about 20% of all Americans suffer from hypertension, which is high blood pressure. Hypertension is present when the systolic blood pressure is 140 or greater, or the diastolic blood pressure is 90 or greater. While both systolic and diastolic pressures are considered important, the diastolic pressure is emphasized when medical treatment is being considered.
Hypertension is sometimes called a silent killer because it may not be detected until a stroke or heart attack occurs. It has long been thought that a certain genetic makeup might account for the development of hypertension. Now researchers have discovered two genes that may be involved in some individuals. One gene codes for angiotensinogen, the plasma protein mentioned previously. Angiotensinogen is converted to a powerful vasoconstrictor in part by the product of the second gene. Persons with hypertension due to overactivity of these genes might one day be cured by gene therapy.
At present, however, the best safeguard against developing hypertension is to have regular blood pressure checks and to adopt a lifestyle that lowers the risk of hypertension.
Stroke and Aneurysm Various cardiovascular diseases- myocardial infarction, stroke, and aneurysm- are associated with hypertension and atherosclerosis. A cerebrovascular accident (CVA), also called a stroke, often results when a small cranial arteriole bursts or is blocked by an embolus. Lack of oxygen causes a portion of the brain to die, and paralysis or death can result. A person is sometimes forewarned of a stroke by a feeling of numbness in the hands or the face, difficulty in speaking, or temporary blindness in one eye.
Aneurysm (expansion of the blood vessel wall into a “sac”) weakens blood vessels, possibly causing them to burst. Aneurysms are most often seen in the abdominal artery or in the arteries leading to the brain. Atherosclerosis and hypertension can weaken the wall of an artery to the point that an aneurysm develops. If a major vessel such as the aorta should burst, death is likely. It is possible to replace a damaged or diseased portion of a vessel with a plastic tube. Cardiovascular function is preserved, because exchange with tissue cells can still take place at the capillaries.
Congestive Heart Failure
In congestive heart failure, a damaged left side of the heart fails to pump adequate blood, and blood backs up in the pulmonary circuit. Therefore, pulmonary blood vessels have become congested. The congested vessels leak fluid into tissue spaces, causing pulmonary edema. The result is shortness of breath, fatigue, and a constant cough with pink, frothy sputum. Treatment consists of the three Ds: diuretics (to increase urinary output), digoxin (to increase the heart’s contractile force), and dilators (to relax the blood vessels). If necessary, a heart transplant is done.
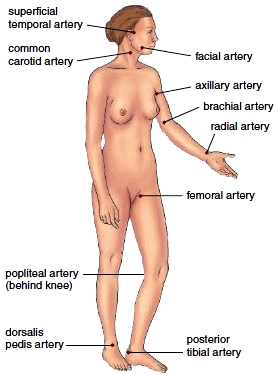
Figure 12.16 The pulse rate. Pulse points are the locations where the pulse can be taken. Each pulse point is named after the appropriate artery.
Figure 12.17 Use of a sphygmomanometer. The technician inflates the cuff with air, gradually reduces the pressure, and listens with a stethoscope for the sounds that indicate blood is moving past the cuff in an artery. This is systolic blood pressure. The pressure in the cuff is further reduced until no sound is heard, indicating that blood is flowing freely through the artery. This is diastolic pressure.